Occipital fixation techniques and complications
Introduction
Occipital fixation techniques and complications
The craniocervical junction (CCJ) functions to both stabilize the neural structures within its central canal as well as allow for a significant amount of cephalic range of motion. Due to the devastating consequences of neurological or ligamentous/skeletal injury at this level, it is crucial to accurately diagnose pathology of the CCJ. An understanding of the unique anatomical relationships is crucial to identifying and reconstructing the unstable CCJ.
Anatomical review of the CCJ
C1, C2 and the occipital bone make up the bony elements that articulate to form the structural foundation of the CCJ. The atlanto-occipital joints are primarily involved in rotational movement. The superior articular surfaces of C1 interface with the occipital condyles in the atlanto-occipital joints and are involved predominantly in flexion-extension movement.
A series of membranes and ligaments adorn the axis, atlas and basal surface of the occipital bone in order to provide stability and freedom of movement to the construct and protection for the nearby neural elements. From a biomechanical perspective, the ligamentous attachments most essential for stable motion at the CCJ are the transverse and alar ligaments, which must be evaluated via magnetic resonance imaging (MRI) if injury is suspected (1,2).
Surgical indications at the CCJ
Many of the most common indications for surgery at the CCJ are due to traumatic injury to the bones or ligaments that ensure stability. Common among these indications are atlanto-occipital or atlantoaxial dislocations, as well as fractures of the occipital condyles, atlas, odontoid, or the ring or body of C2.
Benign and malignant neoplasms account for a wide variety of surgical indications in the CCJ. Extradural tumors, most commonly spinal metastases, can affect the integrity of the bony and soft tissue supporting elements of the spine and are common causes of CCJ instability. Secondary metastases account for 90% of extradural spinal tumors (3). Primary tumors of the CCJ are largely similar to those found in the subaxial spine, although there is an increased prevalence of chordoma, a malignant tumor of remnant cells of the primitive notochord (4). Intradural tumors, while not associated with instability, often cause cord compression at the CCJ requiring decompression and reconstruction. The most common intradural tumors of the spinal canal are meningiomas and nerve sheath tumors (5). Although relatively uncommon, intramedullary lesions such as ependymomas and gliomas can also be found at the CCJ.
Rheumatoid disease is commonly associated with CCJ instability due to inflammation and pannus formation leading to boney erosion and ligamentous laxity. The most common forms of instability in rheumatoid arthritis (RA) are due to atlantoaxial subluxation and cranial settling, also known as basilar invagination, in which the odontoid telescopes through the foramen magnum, compressing neural elements (6). Early intervention is preferred in RA patients due to poorer outcomes once myelopathy develops.
Other indications for surgery at the CCJ include pyogenic infections and congenital and degenerative processes. Pyogenic osteomyelitis affects the CCJ infrequently, but may stem from orofacial infections or as a complication of oral surgeries (7). A variety of congenital abnormalities of the CCJ have been reported, and while they may be found incidentally, many can result in dynamic instability or basilar invagination causing neurologic deficit.
Patients with craniocervical pathology may present with simple neck pain, but symptoms can commonly be correlated with the etiology. Occipital headache is common from laterally compressive masses that impinge upon the occipital nerve roots exiting between C1 and C2. Anterior or posterior cord compression can lead to progressive myelopathy with paresthesias, weakness, and sphincter dysfunction. Compression of the anterior brain stem can produce nystagmus, dysmetria or ataxia. If the inferior cranial nerves are affected, the patient may present with dysphagia, tongue atrophy, or speech deficits. Unstable pathology may be slowly progressive and be rapidly exacerbated by mild trauma.
Imaging and craniometric parameters
Several craniometric parameters have been described to assess the proper alignment at the CCJ. The Wackenheim clivus line, drawn inferiorly down the posterior surface of the clivus, should pass posterior to the odontoid process, but will cross the odontoid in an anterior atlantoaxial dislocation or basilar invagination (8). The McRae and Chamberlain lines define how high the dens should normally reach towards the foramen magnum (9,10).
Several different systems for assessing CCJ integrity have been developed specifically for the assessment of trauma patients. The Powers ratio, calculated as the ratio between a line joining the opisthion and anterior arch of C1 divided by a line joining the basion and posterior arch of C1, should be less than 1 in normal alignment (11). The “Rule of Twelve” states that the basion-dental interval (BDI) and basion-axial interval, the distance between the basion and the posterior axial line, should not exceed 12 mm in adults (12).
Treatment paradigms
Operative management of CCJ pathologies depends on the reducibility of the occiput-atlantoaxial abnormalities. Irreducible lesions usually arise from compressive diseases or fixed instability. Compressive craniocervical pathologies cause a mass effect on the brainstem and/or upper cervical spinal cord. Extension of the head may contuse the critical neural elements with a subsequent neurological deficit on physical examination. In other irreducible lesions, long-standing spinal deformity from, for example, advance-staged autoimmune or degenerative diseases may fixate the head in an immobile position. This uncomfortable posture restricts cephalic kinesthesis. All of these situations warrant a surgical decompression of the CCJ.
Craniocervical fixation should be considered for non-compressive diseases that are reducible: normal spinal alignment easily restored without compromising neurological function. Evaluation of reduction includes preoperative traction or positioning on the operating room table. Preoperative traction entails a harness strapped around the chin or a circular bar with skull tongs. Either system is attached to subsequent weights up to 12 to 15 lbs in adults. X-rays or MRIs under traction can then evaluate for restoration of spinal alignment. Advantages of preoperative traction include patient feedback in real time. Intractable neck pain or, in unusually extreme circumstances, acute neurological deficit with subsequent weights means an irreducible CCJ. Conversely, intraoperative assessment with patient positioning on the operating room table requires fluoroscopy to confirm alignment after reduction under general anesthesia. Because of the absence of patient response, neuromonitoring (see below) becomes mandatory to ensure that the manipulation does not cause neurological injury.
These reduction techniques should cautiously be implemented into a spine surgeon’s practice. Any concern for brainstem/spinal cord injury with head and neck manipulation automatically renders the pathology irreducible that will require decompression.
Irreducible lesions
The details and variations of decompressive techniques at the CCJ extend beyond the scope of this paper. Briefly though, decompression is adjudicated by the site of the lesion (13). Dorsal masses involve a midline suboccipital craniectomy with upper cervical laminectomy(ies) depending on the vertical extent of the pathology. A lateral approach involves an off-midline suboccipital craniectomy/retrosigmoid approach with appropriate decompression of the posterior cervical bony elements for lateral lesions whose cranial extent reach no higher than the internal auditory meatus. Ventral masses could be addressed with a myriad of approaches: far-lateral (transcondylar or paracondylar) craniectomy (14), endoscopic endonasal approach (15), transcervical extrapharyngeal approach (16), and transoral transpharyngeal approach (17).
Reducible lesions
The most common indication for occipitocervical fixation follows iatrogenic instability from extirpation of the diseased tissue at the cranio-atlantoaxial segment; nonetheless, selected reducible disease processes may just require stabilization without surgical decompression. Several techniques may restore spinal alignment. Surgeons must first decide on external immobilization or internal fixation.
External immobilization
Approximately 5% of patients with reducible pathologies can be treated with external immobilization alone (18). Indications would include destructive infections or traumatic fractures. External immobilization should strongly be considered in the pediatric population or young adults because internal occipitocervical arthrodesis would permanently limit head range of motion.
Patients are placed in a halo vest that attaches to a halo ring (19,20). First, the size of ring selected should provide 1–2 cm of clearance from the head circumference. Fixation of the ring must occur below the equator of the skull. The two anterior screws are fastened into the skull 1 cm above the lateral one-third of the eyebrows, and the two posterior screws are placed 5 cm posterior to the top of the tragus. Ideally, the ipsilateral anterior screw remains in-line with the contralateral posterior screw. Opposing screws can then be tightened sequentially for a maximum torque of 0.56 kg/cm3. As a rule-of-thumb, securing the pins finger-tight will collectively generate 4 lb of torque for the sum of all 4 pins. Next, attachment of the halo ring to the halo vest with a construct of vertical rods will fix the head into the desired position. Because the vertical rods will determine the extent of reduction, some surgeons may opt to first connect the halo ring to a pulley with the patient in the supine position. The pulley system attaches to the halo ring on one end and to a 6 kg weight on the other end. Weights are continuously added until a maximum of 15 kg or a desired spinal alignment (whichever comes first). Roentgenography confirms the change in reduction after each subsequent weight. After appropriate reduction, the vertical rod construct fixes the halo ring to the halo vest. Vertical rods must remain symmetrical and parallel for optimal stability. Duration of external immobilization depends on the pathology and the surgeon; regardless, serial images are required to confirm occipitocervical reduction. If necessary, surgeons may increase the angle of head extension after each serial image to maximize the angle of reduction.
The most common complication of external immobilization involves screw loosening (19). Manufacturers suggest re-torquing the screws after 24 hours, 3 days, then weekly until discontinuation of the halo vest. Screw site infections may also occur. Circumventing this nuisance requires basic precautions, like disinfecting the pin site prior to halo ring placement followed by daily cleaning of the insertion sites.
Internal fixation
Internal occipitocervical arthrodesis should be considered in patients who failed to reduce on external fixation or who require stabilization after decompression. Despite the evolution of techniques, the basic approach (exposure) of the procedure remains relatively unchanged. After intubation with general anesthesia, neuromonitoring, which can be at the discretion of the surgeon, should include somatosensory evoked potentials and motor evoked potentials. The patient is positioned prone using a 3-point Mayfield head holder using a standard operating room table with chest bolsters for support. The head should be maintained in a neutral to slightly flexed position, as the patient’s head will be permanently fixed in this position. If the head is fixed in an extended position, the patient may have difficulty with downward gaze, which could lead to difficulties with walking. After appropriate positioning, the incision should extend to the inion. Splitting of the occipital muscles should respect the avascular median raphe. The three muscular layers (outer fascia of the trapezius and sternocleidomastoid; the middle layer with semispinalis capitis, spinalis capitis, and longissimus capitis; and the suboccipital trial bounded by the rectus capitis and obliquus capitis) should not be incised to prevent unnecessary bleeding. The rostral attachment of the occipital muscles should not be removed from the superior nuchal line in order to prevent muscle atrophy or posterior neck disfigurement. Subperiosteal dissection of the muscle off the bone may expose large periosteal bleeders. Bone wax should be immediately applied to decrease the likelihood of air emboli. Soft tissue near the foramen magnum warrants careful exposure with blunt dissection. Electrocautery, if continued at this region, may pose the risk of a durotomy where the thecal sac lacks bony protection at the O-C1 junction.
Spine surgeons should also be cognizant of the vertebral artery that courses as close as 1.5 cm from the C1 midline to enter the foramen magnum in the suboccipital triangle (21). Copious dark blood from the suboccipital venous plexus and the plexus around the vertebral arteries should not be confused with injury to the V3 itself, whose bright red bleeding and strong pulsatility has a very pronounced affect.Now let’s discuss different internal fixation techniques.
Wire fixation
With the development of modern occipital-plate/screw rod systems, the use of wiring is much less prevalent in current practice. However, there are certain indications where wiring may prove useful and should remain a part of the surgeon’s armamentarium. In the pediatric population, inflexible rods and screws may not be suitable in all cases as the hardware may be too large for appropriate bony purchase at the CCJ, and ossification centers may be disrupted. Alternatively, wiring with bone graft affords a semi-rigid construct that does not inhibit continued growth. The autograft is harvested from either the rib or iliac crest. Rib grafts are preferable because of the lower morbidity, higher cortical-cancellous ratio, and less painful donor site. Two suboccipital burr holes are drilled lateral to the bone graft. The dura is separated from the burr hole down to the foramen magnum. Wires that are passed from the burr holes to the foramen magnum are tied around the bone graft for fixation. Postoperatively, children are placed in a halo vest for at least 4–6 months until bony arthrodesis occurs.
Wiring can also play a role in the surgical management of adult patients. Here, rods instead of large bone substitutes attach to wires in patients with a high morbidity burden, such as RA in the elderly. The ease of wires and rods in conjunction with a larger margin of error equates to shorter operative times. Another important advantage includes wider suboccipital craniectomies, which may be warranted in the adult population with advanced-stage disease.
In 1993, Sonntag and Dickman advocated for bending the rod into a U shape (22). The 90° bends rest on the occipital bone, and the free ends attach to the cervical vertebrae. On the occiput, small burr holes are drilled on each side of the rod, and wires are threaded through the burr holes. Braided or twisted wires are beneath the lamina. If the posterior elements have been removed, the wires are alternatively passed around the facet. The tied wires are covered by autograft harvest from the iliac crest. Wire securing is also reinforced by a 5/32-in threaded Steinmann pin. Fusion rates reached 89% with this technique.
Also in 1993, Fehlings et al. published a 93% success rate with a similar technique in which a 5-mm malleable rod was secured to the occipital bone with wires passed through the two burr holes adjacent to the rod. However, segmental fixation to the cervical spine was accomplished by Wisconsin interspinous wires, occasionally supplemented with sublaminar wires (23).
In 2003, the Ohio Medical Instruments Loop was evaluated in a multicenter study (24). The “U” loop of the rod was converted to a low-profile horseshoe to allow for screw or wire/cable fixation. The two descending, knurled rods allow for fixation with screws or wires. Screws were connected with lateral mass linkages. The system reported fusion rates upwards of 96%.
Still yet, Malcolm et al. promised 100% fusion with a Hartshill-Ransford contoured loop (25). The rod is contorted to the angle of the CCJ, and the rod is secured to the suboccipital region in 1 of 2 ways. The wires are threaded one burr hole rostrally and the foramen magnum caudally (Figure 1A,B), or the wires are threaded through burr holes on each side of the rod (Figure 1C,D,E) (26).
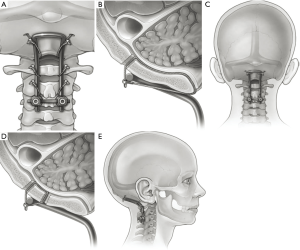
Screw systems
Fusion constructs have evolved significantly since Forrester described the occipitocervical fusion in 1927 with fibular onlay graft (27,28). Modifications of the arthrodesis techniques have sought to generally increase construct rigidity by experimenting with more tensile metals, like titanium (29). One of the initial movements away from wiring techniques occurred at the end of the 20th century when inflexible rods secured by screws promised to limit postoperative external fixation that was often described with prior techniques (30). These novel fusion constructs usually included a plating system to secure the rostral construct to the occipital bone, which promised fewer hardware failures and higher fusion rates (18,31). Variations of these titanium systems exist. In 1999, Pait et al. described the “inside-outside” technique, in which a screw with a long plating system is connected from the occipital bone down to the posterior cervical elements (32). The construct was intended to decrease screw pullout because the suboccipital portion of the bone has an inverted screw placed into a burr hole. Vale et al. attempted to decrease screw pullout with a similar system that utilizes a “T-plate” device (33). In place of rods, an elongated plate attaches to the occipital keel superiorly and to the lateral mass in the spine. One of the most common titanium hardware systems included a Y-shaped plating system that was adopted from orthopaedic long-bone fractures. In the authors’ practice, this is the preferred method for occipital-cervical fixation (Figure 2).
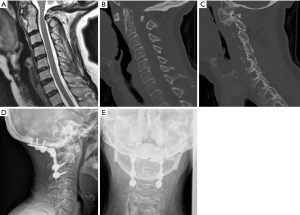
Gentle distraction of the head can also be done in cases where cranial settling is present (34). Depending on the pathology, it may be necessary to tape the shoulders down in order to maximize visualization with lateral fluoroscopy. In addition, if a rib autograft is desired, a mid-thoracic level is typically used and should be prepped and draped out separately in addition to the midline cervical incision. In our practice, we typically will cut a hole in the outer drape to access this area once the hardware has been placed. Bed positioning with reverse-Trendelenburg can be helpful in facilitating hardware placement, but this also raises the possibility of air embolism with significant venous bleeding.
After lateral intraoperative images confirm alignment of the CCJ, the plate is centered at the occipital keel. In the authors’ technique, once the proposed placement of the occipital plate is finalized, pilot holes for the occipital screws through the cortical bone are made using a high-speed matchstick burr. Then, using a power drill, the rest of the screw path is cannulated with the use of a drill guide. Preoperative computed tomography scans can be helpful for planning and sizing appropriate screw length. Depending on the patient anatomy, we advocate at least a 10-mm length screw, and often 12- or 14-mm screws can be used. A ball-tipped feeler can be used to palpate the bottom of the hole to see if there is cortical bone remaining versus dura. In the event of an unintended durotomy with visible egress of cerebral spinal fluid (CSF), no additional repair is required beyond placement of the screw. Bone wax can be used to temporize the leak if there is significant flow which may obscure the surgical field. Maximizing screw length in the occipital keel maximizes bony purchase and ensures construct rigidity. In the pediatric population, there is often times a persistent dural venous sinus in the midline. It is of the utmost importance to avoid lacerating this structure as significant bleeding can result. Careful preoperative planning in such cases is paramount to avoiding such surgical morbidity. In addition, most modern occipital plates have lateral screw holes available to stabilize against rotation forces on the occipital plate. The thickness of the occipital bone here is thinner compared to the keel, and we recommend 6- to 8-mm length screws.
Once the size of the occipital plate screws has been confirmed, the hole is tapped and the screw is inserted. While initial constructs consisted of an occipital bone plate connected to the cervical rods, modern plates now have screws on the lateral plate to accommodate pre-bent or jointed rods. We do not recommend the bending of a straight rod to accommodate the occipitocervical junction as this contributes to metal fatigue, and the gap between the occiput and the most proximal cervical fixation is an area highly susceptible to rod fracture. The distal aspect of the rod must be affixed with posterior cervical spine screws, which in some cases can lead to difficulty with rod placement. Our protocol is to place the occipital plate first, which allows for alignment of distal screws in line to facilitate rod capture.
Although there have been reports of OC fusion only down to C2 as a stopping point, we typically advocate a minimum stopping point of C3 or C4 for distal fixation (Figure 2) (31). Depending on the patient’s anatomy one can consider including C1 lateral mass screws to the construct. However, in most cases the position of the C1 lateral mass screw head in comparison to the occipital plate and C2 screws can sometimes translate to an inability to connect the C1 screw to the rod (35). There have been reports of failure of the occipitocervical fusion with distal fixation only at the C2 pedicle, pars, or lamina without incorporating C1 (35). With the advent of off-sets, however, C1 screws may attach to the construct without compromising fusion rates if one is considering stopping at C2 (36,37). For C2 screw placement, we typically utilize pars screws, although laminar screws and pedicle screws are also options for fixation. In the subaxial spine (C3 to C7), lateral mass screws are used. If the pathology necessitates crossing the cervical thoracic junction, pedicle screws are placed in the upper thoracic area without placing C7 lateral mass screws. In such long constructs, the medial lateral alignment of the screw heads may complicate rod placement. However, screw systems that feature cross-connectors, or extended medial-lateral angulation/translation of the screw head can help facilitate rod placement.
Hooks
In 2002, Faure described the hook technique (38). Burr holes are placed in the squamous portion of the occipital bone: 2 cm from the midline and 3 cm below the superior nuchal line. The dura is dissected away from the inner table. Two occipital hooks are placed back-to-back (Figure 3), and the rod is threaded through the duplicated hooks. The caudal end of the rod attaches to cervical laminar hooks. Alternatively, Paquis et al. suggested cervical interlaminar claws (39). The rod is contoured to align with the curvature of the occipitocervical junction, and set screws secure the rod in place. When the occipital bone exceeds 7 mm in thickness, occipital hooks are replaced with occipital screws (27). Hooks alternatively latch onto the foramen magnum to create a “claw construct.”
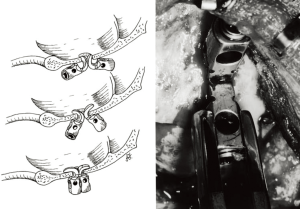
C1-occipital condyle screw
Gonzales et al described a transarticular screw at the craniovertebral junction for occipital atlantoaxial instability (40). The occipitoaxial joints are fused under guidewire and fluoroscopic techniques with the transarticular screws placed starting at the midpoint of the C1 lateral mass with a trajectory 10–20° medially and directed superiorly towards the middle aspect of the occipital condyle. The screw lengths range between 4 mm × 22–33 mm, ending just caudal to the hypoglossal canal. Biomechanical studies have reported promising results with higher fusion rates and less flexion-extension motion. However, surgeons should recognize that screw and plated fusions pose less of a risk to patients with respect to violation into the canal and injury to critical neurovascular structures. Details of this technique will be discussed in a different article.
Complications
As with any surgical technique there are potential complications that can arise. The most critical involve injury to key neurological and vascular structures. In placement of the occipital plate unintended durotomies may occur causing an egress of CSF. Typically, placement of the screw is all that is necessary to stop the leak, but in cases with high flow leaks a plug of bone wax can be used to temporize the leak. If damage to the underlying cerebellar cortex is suspected, urgent CT scanning should be performed to rule out a suboccipital hematoma. Such lesions are potentially fatal due to the limited space of the posterior fossa, and if such an injury is identified, emergent neurosurgical intervention will be necessary. In patients with severe spinal cord or brain stem compression, intraoperative neuromonitoring with somatosensory and motor evoked potentials is recommended. In many cases, positioning may compromise compressed structures, and baseline motor evoked potentials are recommended prior to flipping the patient supine. Clear communication with anesthesia to avoid hypotension in such cases is highly recommended.
For vascular injuries, injury to the vertebral artery carries the most significant consequences. Care should be used to avoid monopolar cautery when exposing the C1 arch and foramen magnum. An injury can occur either during exposure or placement of hardware. In the case of an arterial injury, control can be obtained by applying direct pressure with a cotton patty while trying to localize the injury. In some cases, a direct repair may be possible, but in the event that a repair is not possible ligation of the vessel may be necessary. If an injury occurs and the contralateral side has not yet been instrumented, the operation should be terminated so that a vascular study, preferably catheter angiography with digital-subtraction, can be performed to assess the vertebral artery anatomy. Bilateral injury to the vertebral artery is potentially fatal, and must be avoided at all costs. In addition, in rare cases where surgical hemostasis of a vertebral artery injury cannot be obtained, endovascular occlusion of the vertebral artery is also an option with access via the popliteal artery in the prone position.
Significant venous bleeding can also be encountered, as there is a substantial venous plexus between C1 and C2. This is typically encountered during exposure. We advocate the judicious use of an injectable hemostatic agent (Floseal or Surgi-Flo) in combination with cotton patties to tamponade the venous hemorrhage, and moving to the contralateral side to work. Rarely a pulmonary air embolism may occur, in which case the surgical field should be copiously irrigated. Occasionally, due to pulmonary or hemodynamic instability a patient may have to be turned emergently supine to allow for resuscitation or other interventions. Clear communication with the anesthesia team in such cases is paramount to prevent a catastrophic complication.
Finally, hardware complications and pseudoarthrosis occur typically in delayed fashion. In such cases, it is important to ascertain the biomechanical cause for failure so that the revision can be tailored accordingly. In many cases, poor bone quality is an underlying culprit for failure, and the additional use of external fixation, even with a halo vest, may be indicated. If not used in the index procedure, rib allograft may help improve the chances of a successful fusion. In addition, there are a number of biological products designed to promote fusion, which can be used as an adjunct. In many cases, these may be off-label uses of such products at the occipital-cervical junction and the surgeon may have to make a judgment on a case-by-case basis.
Acknowledgments
Funding: None.
Footnote
Provenance and Peer Review: This article was commissioned by the Guest Editors (Lee A. Tan and Ilyas S. Aleem) for the series “Advanced Techniques in Complex Cervical Spine Surgery” published in Journal of Spine Surgery. The article was sent for external peer review organized by the Guest Editors and the editorial office.
Conflicts of Interest: The series “Advanced Techniques in Complex Cervical Spine Surgery” was commissioned by the editorial office without any funding or sponsorship. V Chang receives research funding from Medtronic, who was not involved in this project, specifically. He is also a consultant for Globus Medical, K2M, and SpineGuard. The other authors have no conflicts of interest to declare.
Ethical Statement: The authors are accountable for all aspects of the work to ensure that questions related to the accuracy or integrity of any part of the work are appropriately investigated and resolved.
Open Access Statement: This is an Open Access article distributed in accordance with the Creative Commons Attribution-NonCommercial-NoDerivs 4.0 International License (CC BY-NC-ND 4.0), which permits the non-commercial replication and distribution of the article with the strict proviso that no changes or edits are made and the original work is properly cited (including links to both the formal publication through the relevant DOI and the license). See: https://creativecommons.org/licenses/by-nc-nd/4.0/.
References
- Panjabi M, Dvorak J, Crisco J 3rd, et al. Flexion, extension, and lateral bending of the upper cervical spine in response to alar ligament transections. J Spinal Disord 1991;4:157-67. [Crossref] [PubMed]
- Dvorak J, Schneider E, Saldinger P, et al. Biomechanics of the craniocervical region: the alar and transverse ligaments. J Orthop Res 1988;6:452-61. [Crossref] [PubMed]
- Simmons ED, Zheng Y. Vertebral tumors: surgical versus nonsurgical treatment. Clin Orthop Relat Res 2006.233-47. [Crossref] [PubMed]
- Boriani S, Bandiera S, Biagini R, et al. Chordoma of the mobile spine: fifty years of experience. Spine (Phila Pa 1976) 2006;31:493-503. [Crossref] [PubMed]
- Schellinger KA, Propp JM, Villano JL, et al. Descriptive epidemiology of primary spinal cord tumors. J Neurooncol 2008;87:173-9. [Crossref] [PubMed]
- Bouchaud-Chabot A, Liote F. Cervical spine involvement in rheumatoid arthritis. A review. Joint Bone Spine 2002;69:141-54. [Crossref] [PubMed]
- Gormley W, Rock J. Spontaneous atlantoaxial osteomyelitis: no longer a rare case? Case report. Neurosurgery 1994;35:132-5; discussion 135-6. [Crossref] [PubMed]
- Wackenheim A. Radiologic diagnosis of congenital forms, intermittent forms and progressive forms of stenosis of the spinal canal at the level of the atlas. Acta Radiol Diagn (Stockh) 1969;9:759-68. [PubMed]
- McRae DL, Barnum AS. Occipitalization of the atlas. Am J Roentgenol Radium Ther Nucl Med 1953;70:23-46. [PubMed]
- Chamberlain WE. Basilar Impression (Platybasia): A Bizarre Developmental Anomaly of the Occipital Bone and Upper Cervical Spine with Striking and Misleading Neurologic Manifestations. Yale J Biol Med 1939;11:487-96. [PubMed]
- Powers B, Miller MD, Kramer RS, et al. Traumatic anterior atlanto-occipital dislocation. Neurosurgery 1979;4:12-7. [Crossref] [PubMed]
- Harris JH Jr, Carson GC, Wagner LK. Radiologic diagnosis of traumatic occipitovertebral dissociation: 1. Normal occipitovertebral relationships on lateral radiographs of supine subjects. AJR Am J Roentgenol 1994;162:881-6. [Crossref] [PubMed]
- Bruneau M, George B. Classification system of foramen magnum meningiomas. J Craniovertebr Junction Spine 2010;1:10-7. [Crossref] [PubMed]
- Lanzino G, Paolini S, Spetzler RF. Far-lateral approach to the craniocervical junction. Neurosurgery 2005;57:367-71; discussion 367-71. [PubMed]
- Liu JK, Patel J, Goldstein IM, et al. Endoscopic endonasal transclival transodontoid approach for ventral decompression of the craniovertebral junction: operative technique and nuances. Neurosurg Focus 2015;38:E17. [Crossref] [PubMed]
- Wolinsky JP, Sciubba DM, Suk I, et al. Endoscopic image-guided odontoidectomy for decompression of basilar invagination via a standard anterior cervical approach. Technical note. J Neurosurg Spine 2007;6:184-91. [Crossref] [PubMed]
- Dlouhy BJ, Dahdaleh NS, Menezes AH. Evolution of transoral approaches, endoscopic endonasal approaches, and reduction strategies for treatment of craniovertebral junction pathology: a treatment algorithm update. Neurosurg Focus 2015;38:E8. [Crossref] [PubMed]
- Quinones-Hinojosa A. Schmidek and Sweet: Operative Neurosurgical Techniques 2-Volume Set: Indications, Methods and Results (Expert Consult-Online and Print). Elsevier Health Sciences; 2012.
- Aebi M, Arlet V, Webb JK. AOspine manual. Thieme; 2007.
- Halo Vest. In: Occipitocervical Trauma - Odontoid, AAII Halo vest. AO Foundation. Available online: https://www2.aofoundation.org/wps/portal/!ut/p/a1/04_Sj9CPykssy0xPLMnMz0vMAfGjzOKN_A0M3D2DDbz9_UMMDRyDXQ3dw9wMDAzMjYEKIvEocDQnTr8BDuBoQEh_QW5oKAD4ENaS/dl5/d5/L2dJQSEvUUt3QS80SmlFL1o2XzJPMDBHSVMwS09PVDEwQVNFMUdWRjAwMDcz/?bone=Spine&classification=51-Odontoid%2C%20AAII&method=Halo%20vest&segment=TraumaUpperCervical&showPage=redfix&treatment=Operative
- Posterior access to C1-C2. In: Occipitocervical trauma. AO Foundation. Available online: https://www2.aofoundation.org/wps/portal/!ut/p/a1/04_Sj9CPykssy0xPLMnMz0vMAfGjzOKN_A0M3D2DDbz9_UMMDRyDXQ3dw9wMDAzMjYEKIvEocDQnTr8BDuBoQEh_QW5oKAD4ENaS/dl5/d5/L2dJQSEvUUt3QS80SmlFL1o2XzJPMDBHSVMwS09PVDEwQVNFMUdWRjAwMDcz/?approach=Posterior%20access%20to%20C1-C2&bone=Spine&segment=TraumaUpperCervical&showPage=approach&contentUrl=/srg/51/04-Approaches/A200_PostToC1C1.jsp
- Sonntag VK, Dickman CA. Craniocervical stabilization. Clin Neurosurg 1993;40:243-72. [PubMed]
- Fehlings MG, Errico T, Cooper P, et al. Occipitocervical fusion with a five-millimeter malleable rod and segmental fixation. Neurosurgery 1993;32:198-207; discussion 207-8. [Crossref] [PubMed]
- Singh SK, Rickards L, Apfelbaum RI, et al. Occipitocervical reconstruction with the Ohio Medical Instruments Loop: results of a multicenter evaluation in 30 cases. J Neurosurg 2003;98:239-46. [PubMed]
- Malcolm GP, Ransford AO, Crockard HA. Treatment of non-rheumatoid occipitocervical instability. Internal fixation with the Hartshill-Ransford loop. J Bone Joint Surg Br 1994;76:357-66. [Crossref] [PubMed]
- Klimo P Jr, Astur N, Gabrick K, et al. Occipitocervical fusion using a contoured rod and wire construct in children: a reappraisal of a vintage technique. J Neurosurg Pediatr 2013;11:160-9. [Crossref] [PubMed]
- Vender JR, Rekito AJ, Harrison SJ, et al. The evolution of posterior cervical and occipitocervical fusion and instrumentation. Neurosurg Focus 2004;16:E9. [Crossref] [PubMed]
- Frankel BM, Hanley M, Vandergrift A, et al. Posterior occipitocervical (C0-3) fusion using polyaxial occipital condyle to cervical spine screw and rod fixation: a radiographic and cadaveric analysis. J Neurosurg Spine 2010;12:509-16. [Crossref] [PubMed]
- Garrido BJ, Myo GK, Sasso RC. Rigid versus nonrigid occipitocervical fusion: a clinical comparison of short-term outcomes. J Spinal Disord Tech 2011;24:20-3. [Crossref] [PubMed]
- Grob D, Dvorak J, Panjabi MM, et al. The role of plate and screw fixation in occipitocervical fusion in rheumatoid arthritis. Spine (Phila Pa 1976) 1994;19:2545-51. [Crossref] [PubMed]
- Hurlbert RJ, Crawford NR, Choi WG, et al. A biomechanical evaluation of occipitocervical instrumentation: screw compared with wire fixation. J Neurosurg 1999;90:84-90. [PubMed]
- Pait TG, Al-Mefty O, Boop FA, et al. Inside-outside technique for posterior occipitocervical spine instrumentation and stabilization: preliminary results. J Neurosurg 1999;90:1-7. [PubMed]
- Vale FL, Oliver M, Cahill DW. Rigid occipitocervical fusion. J Neurosurg 1999;91:144-50. [PubMed]
- Abumi K, Takada T, Shono Y, et al. Posterior occipitocervical reconstruction using cervical pedicle screws and plate-rod systems. Spine (Phila Pa 1976) 1999;24:1425-34. [Crossref] [PubMed]
- Shad A, Shariff SS, Teddy PJ, et al. Craniocervical fusion for rheumatoid arthritis: comparison of sublaminar wires and the lateral mass screw craniocervical fusion. Br J Neurosurg 2002;16:483-6. [Crossref] [PubMed]
- Gunnarsson T, Massicotte EM, Govender PV, et al. The use of C1 lateral mass screws in complex cervical spine surgery: indications, techniques, and outcome in a prospective consecutive series of 25 cases. J Spinal Disord Tech 2007;20:308-16. [Crossref] [PubMed]
- Garrido BJ, Puschak TJ, Anderson PA, et al. Occipitocervical fusion using contoured rods and medial offset connectors: description of a new technique. Orthopedics 2009. [Crossref] [PubMed]
- Faure A, Monteiro R, Hamel O, et al. Inverted-hook occipital clamp system in occipitocervical fixation. Technical note. J Neurosurg 2002;97:135-41. [PubMed]
- Paquis P, Breuil V, Lonjon M, et al. Occipitocervical fixation using hooks and screws for upper cervical instability. Neurosurgery 1999;44:324-30; discussion 330-1. [Crossref] [PubMed]
- Gonzalez LF, Crawford NR, Chamberlain RH, et al. Craniovertebral junction fixation with transarticular screws: biomechanical analysis of a novel technique. J Neurosurg 2003;98:202-9. [PubMed]