The Barrow Biomimetic Spine: effect of a 3-dimensional-printed spinal osteotomy model on performance of spinal osteotomies by medical students and interns
Introduction
In an era of resident training characterized by duty-hour restrictions and increased oversight in the operating room, there has been growing concern about the most effective way to train residents while maintaining patient safety and improving surgical outcomes. Recent studies have shown that restrictions on duty hours have had a significant impact on the quality of resident training in terms of academic productivity, board examination scores, and the number of hours spent in the operating room (1,2). In the presence of these new constraints, it is becoming increasingly clear that the traditional Halstedian model of surgical mentorship must be supplemented to allow residents sufficient opportunity to master complex surgical procedures without extending the length of residency or exceeding work-hour restrictions.
In recent years, there has been growing interest in the use of simulation-based surgical training to supplement time in the operating room. Surgical simulation allows trainees to focus on mastering the individual steps and technical skills required to perform complex surgical procedures in a controlled, risk-free setting before attempting them in the high-stakes environment of the operating room (3-5). Several studies have shown that both resident and attending physicians have strongly positive attitudes toward simulation training for its potential to supplement time in the operating room, to provide objective assessments of surgical skill, and to improve patient outcomes (3,6).
One simulation modality that has been received with significant enthusiasm is 3-dimensional (3D) printing (7). 3D-printed models have found increasing use in a diverse array of neurosurgical subspecialties, with models ranging from physical replicas of vascular networks to aid with preoperative anatomical visualization (8,9) to multistep, patient-specific screw guides to assist with pedicle screw insertion (10). The use of 3D-printed models in resident education holds several advantages over traditional cadaver labs, including increased anatomical consistency, reduced operating costs, and decreased facility and personnel requirements (11).
In spine surgery, 3D printing has been used for several applications, including teaching spinal anatomy (12,13), planning surgical trajectories (14), and creating intervertebral disc replacements in vitro (15). However, compared to other fields, spine surgery has a relative scarcity of simulation research (16-19). Ongoing studies at Barrow Neurological Institute are aimed at addressing this deficiency by creating a synthetic spine model—the Barrow Biomimetic Spine—capable of replicating the physical properties of cadaveric spines for both resident education and biomechanical testing. This model allows residents to learn complex spinal procedures through hands-on surgical manipulation of a 3D-printed spine replica that closely mimics the physical properties of the human vertebral column.
In this study, we investigated the use of a modified form of the Barrow Biomimetic Spine to teach junior residents and medical students the Schwab osteotomy classification system. The Schwab classification system provides a common vocabulary to describe spinal osteotomies. The purpose of this study was to evaluate the utility of a custom, 3D-printed spine model to help surgical trainees understand and perform the Schwab osteotomy grading scale.
Methods
Institutional review board approval was not required because the research involved a comparison between standardized instructional techniques for neurosurgical trainees, and trainee scores were recorded anonymously by study group. Patient consent was not required because no patients were involved in this study.
Spine models
Fourteen spine models were made for this study. A high-resolution computed tomogram of a normal lumbar spine was converted into an .stl file format using the Materialise Mimics software suite (Materialise, NV, Leuven, Belgium). The L3–L5 segment was obtained from the computed tomogram and uploaded as an .stl file into the Simplify3D (Simplify3D, Blue Ash, Ohio, USA) printing platform. Using a cortical thickness of 4 shells, a cancellous density of 20%, and acrylonitrile butadiene styrene (ABS) plastic filament, we printed 12 white L3–L5 models using a Flashforge Creator Pro 3D printer (Flashforge USA, City of Industry, California, USA). These models were all printed from the same .stl file, so were anatomically identical. Four of these models were painted with 5 different colors, each representing the bone that is to be removed with successive Schwab grade osteotomies (Figure 1). The other 8 models were left plain white, as they were to be used for practical testing of osteotomy performance.
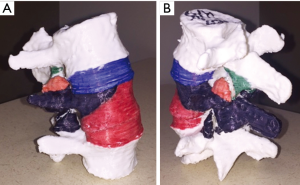
Two additional models were printed using ABS filament of various colors to represent the different osteotomies (Figure 2). These models were used for additional practical testing, and so the colors used to represent certain osteotomies were purposely made different from those used in the 4 painted models.
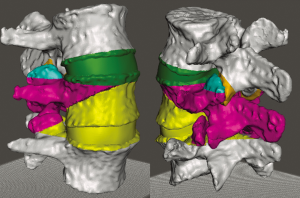
Test population and study design
Eight volunteer trainees participated in this study: 6 were 4th-year medical students completing a sub-internship in neurosurgery at the authors’ home institution, and 2 were interns in neurosurgery who had not yet begun a neurosurgical rotation. Before participating in the study, trainees were asked about their knowledge of the Schwab osteotomy grading system; none of the trainees reported being familiar with this system or with spinal osteotomies in general.
The 8 trainees were randomized into two test groups. Each test group was given a copy of the Schwab et al. article (20) detailing the Schwab osteotomy grading scale, including the 2-dimensional (2D) images used to describe each osteotomy grade. Trainees in group 1 received only these materials. Trainees in group 2 were additionally provided with a painted L3–L5 spine model and a color scheme explaining which osteotomy grade each color represented. All trainees were asked not to discuss the osteotomy grading scale with each other or to seek learning resources outside of those provided.
The trainees were given two evenings to study their respective learning materials and then were administered written and practical examinations to test their understanding and ability to perform spinal osteotomies. The written examination consisted of 8 questions that tested their overall understanding of the Schwab osteotomy grading scale, as well as specific details about each osteotomy (Supplement I). The practical examination required the trainees to perform Schwab grade 2 osteotomies, followed by Schwab grade 4 osteotomies, on a plain white L3–L5 spinal model. The portions of the models that were removed during the practical testing were collected and weighed for quantitative comparison between the two groups.
Statistical analysis
A total of 10 points was achievable in the written and practical examinations, 8 points for the written examination and 2 points for the practical examination. Written examination questions were scored as correct (1 point) or incorrect (0 points). The practical examination tasks were scored as a percentage of 1 point based on the successful removal of specific bone segments for Schwab grades 2 and 4 osteotomies, respectively. For Schwab grade 2 osteotomies, 0.5 points were awarded for successful removal of the inferior articulating processes and another 0.5 points for the successful removal of the superior articulating processes of the tested level. For Schwab grade 4 osteotomies, 0.33 points each were awarded for removal of the inferior level’s facet, the pedicles and bone wedge of the index vertebral body, and the superior disc.
The Wilcoxon rank sum (Mann Whitney) test was applied to compare test scores of groups 1 and 2, as well as the weights of bone removed during Schwab grades 2 and 4 osteotomy testing.
Results
All spine models were successfully printed, and all eight volunteers successfully completed testing following two evenings of study. Each test group contained 3 sub-interns and 1 intern. The mean ± standard deviation (SD) overall test score for group 1 was 6.83±0.45, and the mean test score for group 2 was 9.50±0.69. The mean weight of the model removed during the Schwab grade 2 osteotomy practical test for group 1 was 2,332±603.5 mg, and for group 2 it was 2,200±623.5 mg. The mean weight of the model removed during the Schwab grade 4 osteotomy practical test for group 1 was 11,383±10,858.3 mg, and the mean weight removed for group 2 was 22,161±1,883.0 mg.
The statistical comparison of test group data revealed that group 2 achieved significantly higher written test scores (mean, 7.75±0.50) than group 1 (mean, 5.75±0.50, P=0.023). Group 2 also achieved significantly higher practical test scores (mean, 1.75±0.32) than group 1 (mean, 1.08±0.09, P=0.025). In addition, group 2 achieved higher overall examination scores (mean, 9.5±0.69) than group 1 (mean, 6.83±0.45, P=0.028). Group 2 trainees also removed more bone during Schwab grade 4 osteotomy testing, though the difference did not reach significance (P=0.114). Comparisons of weight removed during Schwab grade 2 osteotomy testing were also not significant (P=0.886). Individual trainee test results and statistical comparisons are summarized in Table 1.
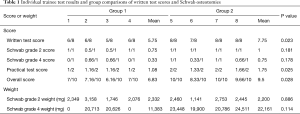
Full table
Discussion
In this study, we showed that the use of a custom, 3D-printed model of the lumbar spine improved learners’ understanding of the Schwab osteotomy grading scale. Our data show that medical students and residents who were given a 3D-printed lumbar spine model in addition to the Schwab et al. article (20) (group 2) performed significantly better than the control group when performing posterior column and 3-column osteotomies. Group 2 students also removed more bone (did not reach significance) in the process of performing a 3-column osteotomy, in addition to scoring significantly higher on the written examination. Notably, there was no significant difference between the 2 groups on the execution of a Schwab grade 2 osteotomy, which requires removal of the superior and inferior articular processes of the index facet joint. This discrepancy could have been because the marginal benefit of the model was masked when learners were tested on simpler anatomical knowledge and didactic concepts and that the advantages of the model became apparent only when learners were tested on the execution of a procedure requiring more extensive knowledge of the 3D spinal anatomy (i.e., a 3-column osteotomy).
Although no qualitative feedback was formally collected during this study, several important comments were made by trainees in both groups. Trainees in both groups 1 and 2 reported after their practical testing that the images in the Schwab et al. article (20) seemed intuitive at first, but when faced with a 3D representation of the osteotomies, they had a much more difficult time envisioning the anatomy. For example, 2 trainees in the control group scored 0/1 on their performance of the Schwab grade IV osteotomy. They both reported after the practical test that they thought they understood the anatomy based on the 2D figures but had trouble mentally converting those 2D images onto a 3D model. Several trainees in group 2 furthermore reported that they spent the majority of their study time focused on correlating the Schwab et al. article (20) images to the supplied 3D models. This advantage of the group 2 trainees likely explains their better performance on the written and practical examinations, and furthermore emphasizes the benefit of 3D imaging and modeling in teaching complex 3D anatomical concepts such as 3-column osteotomies.
3D printing, also known as additive manufacturing, has revolutionized the process of rapid prototyping since its introduction in the 1980s. Physicians and researchers have begun using 3D printers to create physical, 3D models of patient-specific structures that until recently were confined to 2D projections on screens or in textbooks (21-24). Unsurprisingly, this technique has emerged as a topic of enormous interest in the surgical literature in recent years (5,7,11,25). To date, the literature has subdivided the use of 3D printing in surgery into 3 broad categories: (I) patient and resident education; (II) preoperative planning; and (III) patient-specific implant creation (4). The use of 3D-printed models for resident education has become increasingly important in the current era of surgical training (3,5,16). A recent review demonstrated that, as of October 2017, a total of 27 articles had been published to investigate the use of 3D printing in surgical training; of these, 10 articles were neurosurgery specific—the highest number of articles from any single specialty (11).
The use of 3D-printed models has been shown to have a positive impact on surgical resident training in many specialties. Surgical residents and attendings who have been given the opportunity to train on 3D-printed models have rated these models very highly on several metrics, including their ability to aid in the understanding of surgical procedures and anatomy, their similarity to actual cadaveric specimens, and their potential to help trainees improve surgical skills (13,26-36). More objectively, 3D-printed surgical models have been shown to improve the quality of surgical plans for pancreatic cancer (26), the accuracy of renal anatomy assessment for nephrolithotripsy (27), the quality of endoscopic ear surgery skills (28), and the quality of drilling, curetting, and aspirating skills used during endoscopic endonasal surgery (37). A recent study furthermore demonstrated that neurosurgery residents who trained on a 3D-printed cranial model were significantly more accurate when placing external ventricular drains (38). We believe that the results of this study, which demonstrate an improvement in the performance of complex lumbar osteotomies after the use of a 3D-printed lumbar spine for training, adds to the growing body of evidence supporting the utility of 3D printing in surgical education and simulation.
A recent literature review demonstrated that 36 articles were published about 3D printing in neurosurgery between 2012 and 2016 (7). However, of these 36 articles, only 5 dealt with spine surgery specifically, and only 3 articles focused on the use of 3D-printed models for surgical education. Several reports have also pointed out the deficiency of research in surgical simulation in spine surgery relative to the other surgical subspecialties (17-19). The Barrow Biomimetic Spine project, which began in 2015, was established to help address this deficiency. The Barrow Biomimetic Spine has undergone numerous iterations, with the goal of producing a model that most closely resembles a cadaveric vertebral column (39,40). Recent publications originating from the Barrow Biomimetic Spine project have demonstrated that this model is an accurate replication of the cadaveric spine in terms of fundamental biomechanical performance and radiographic qualities. Moreover, because this spine model is created using 3D printers, it has the advantage of allowing for patient-specific customization of each individual model, while also being more cost-efficient and less resource-intensive than a traditional cadaver lab. The material cost of the L3–L5 models used in this study, for example, was less than $5 per model, whereas cadaveric spine segments can cost hundreds to thousands of dollars.
Despite recent enthusiasm for the use of 3D printing in surgical simulation, a few caveats should be considered when considering the landscape of studies that have already been published and when planning future research in the field. Several prior reviews have noted that most papers published on the use of 3D printing in surgical education have relied on surveys of study participants to establish the validity and quality of their models (7,11,25). This low-quality, subjective data, while useful in certain contexts, does not provide the robust, quantitative evidence necessary to establish the true utility of these models. Moreover, it has been suggested that this relatively nascent field is the subject of considerable publication bias, given that almost all articles published on the use of 3D printing in surgical education have reported positive results (11). Finally, it has been suggested that the rush to adopt 3D printing has resulted in the scattered development of many individual institution-specific models—a situation that is neither cost-effective nor efficient (41).
Our study specifically has several limitations that may have affected the strength of our conclusions. The sample size was small and included only 8 participants who were randomized into 2 study groups. Additionally, the study personnel responsible for rating the quality of the participants’ osteotomies were not blinded to the participants’ study groups, which may have introduced a level of confirmation bias. Despite these limitations, we believe that the proposed model represents a valuable resource for teaching the Schwab osteotomy grading system and may serve as a springboard for future uses of the Barrow Biomimetic Spine in surgical education.
Future studies using the Barrow Biomimetic Spine in surgical education may focus on teaching residents other complex surgical and anatomical concepts using customized 3D-printed spine models. For instance, models of the entire cervical, thoracic, and lumbar spine with various forms of scoliosis could be printed and used to help residents understand the Scoliosis Research Society-Schwab scoliosis classification system. In addition, isolated spine segments printed with idiopathic or degenerative pathology could be used to help teach residents how to perform routine procedures, such as foraminotomies and laminectomies, in the presence of distorted spinal anatomy. Overall, we believe that 3D printing represents a new and exciting frontier in surgical simulation and education. We hope that the spine model we have introduced here further reinforces the evidence supporting the use of such models in resident education and surgical planning.
Conclusions
The modified version of the Barrow Biomimetic Spine presented here was an effective adjunct for teaching the Schwab osteotomy grading system to surgical trainees. Fourth-year medical students and first-year residents who were given the 3D model in addition to the grading system article performed significantly better on written and practical assessments than participants who were given the article alone. Notably, there were no differences in performance between the two groups on Schwab grade two osteotomies. We believe that the current study illustrates the didactic value of the Barrow Biomimetic Spine in teaching early surgical residents the Schwab osteotomy grading scale—a surgical concept that requires a thorough understanding of vertebral column anatomy.
Supplement I Written examination
- How many Schwab osteotomy grades are there?
- Which osteotomies are more destabilizing, higher grade or lower grade?
- Which Schwab osteotomy grades require removal of bone from the posterior column only?
- Which grade osteotomy has been performed on this model as provided?
- Which colors should be removed from this model for a Schwab grade 3 osteotomy?
- Which colors should be removed from this model for a Schwab grade 5 osteotomy?
- Which grade osteotomy requires a discectomy at the superior disc level?
- Which grade osteotomy requires a discectomy at the inferior disc level?
Acknowledgements
The authors thank the staff of Neuroscience Publications at Barrow Neurological Institute for assistance with manuscript preparation. This work was supported by Barrow Neurological Foundation and Lisa Family Foundation.
Footnote
Conflicts of Interest: The authors have no conflicts of interest to declare.
References
- Jagannathan J, Vates GE, Pouratian N, et al. Impact of the Accreditation Council for Graduate Medical Education work-hour regulations on neurosurgical resident education and productivity. J Neurosurg 2009;110:820-7. [Crossref] [PubMed]
- McCall T, Rao G, Kestle J. Work hour restrictions: impact on neurosurgical resident training at the University of Utah. AANS Bulletin, 2005;14.
- Ganju A, Aoun SG, Daou MR, et al. The role of simulation in neurosurgical education: a survey of 99 united states neurosurgery program directors. World Neurosurg 2013;80:e1-8. [Crossref] [PubMed]
- Rehder R, Abd-El-Barr M, Hooten K, et al. The role of simulation in neurosurgery. Childs Nerv Syst 2016;32:43-54. [Crossref] [PubMed]
- Selden NR, Origitano TC, Hadjipanayis C, et al. Model-based simulation for early neurosurgical learners. Neurosurgery 2013;73 Suppl 1:15-24. [Crossref] [PubMed]
- Kirkman MA, Muirhead W, Nandi D, et al. Development and psychometric evaluation of the “Neurosurgical Evaluation of Attitudes towards simulation Training” (NEAT) tool for use in neurosurgical education and training. World Neurosurg 2014;82:284-91. [Crossref] [PubMed]
- Randazzo M, Pisapia J, Singh N, et al. 3D printing in neurosurgery: a systematic review. Surg Neurol Int 2016;7:S801-S809. [Crossref] [PubMed]
- Abla AA, Lawton MT. Three-dimensional hollow intracranial aneurysm models and their potential role for teaching, simulation, and training. World Neurosurg 2015;83:35-6. [Crossref] [PubMed]
- Thawani JP, Pisapia JM, Singh N, et al. Three-dimensional printed modeling of an arteriovenous malformation including blood flow. World Neurosurg 2016;90:675-83. [Crossref] [PubMed]
- Sugawara T, Higashiyama N, Kaneyama S, et al. Multistep pedicle screw insertion procedure with patient-specific lamina fit-and-lock templates for the thoracic spine. J Neurosurg Spine 2013;19:185-90. [Crossref] [PubMed]
- Langridge B, Momin S, Coumbe B, et al. Systematic review of the use of 3-dimensional printing in surgical teaching and assessment. J Surg Educ 2018;75:209-21. [Crossref] [PubMed]
- Li Z, Li Z, Xu R, et al. Three-dimensional printing models improve understanding of spinal fracture—a randomized controlled study in China. Sci Rep 2015;5:11570. [Crossref] [PubMed]
- Liew Y, Beveridge E, Demetriades AK, et al. 3D printing of patient-specific anatomy: a tool to improve patient consent and enhance imaging interpretation by trainees. Br J Neurosurg 2015;29:712-4. [Crossref] [PubMed]
- Bova FJ, Rajon DA, Friedman WA, et al. Mixed-reality simulation for neurosurgical procedures. Neurosurgery 2013;73 Suppl 1:138-45. [Crossref] [PubMed]
- Whatley BR, Kuo J, Shuai C, et al. Fabrication of a biomimetic elastic intervertebral disk scaffold using additive manufacturing. Biofabrication 2011;3:015004. [Crossref] [PubMed]
- Bohm PE, Arnold PM. Simulation and resident education in spinal neurosurgery. Surg Neurol Int 2015;6:33. [Crossref] [PubMed]
- Harrop J, Rezai AR, Hoh DJ, et al. Neurosurgical training with a novel cervical spine simulator: posterior foraminotomy and laminectomy. Neurosurgery 2013;73 Suppl 1:94-9. [Crossref] [PubMed]
- Walker JB, Perkins E, Harkey LH. A novel simulation model for minimally invasive spine surgery. Neurosurgery 2009;65:188-95; discussion 195. [PubMed]
- Woodrow SI, Dubrowski A, Khokhotva M, et al. Training and evaluating spinal surgeons: the development of novel performance measures. Spine 2007;32:2921-5. [Crossref] [PubMed]
- Schwab F, Blondel B, Chay E, et al. The comprehensive anatomical spinal osteotomy classification. Neurosurgery 2014;74:112-20; discussion 120. [Crossref] [PubMed]
- Breimer GE, Bodani V, Looi T, et al. Design and evaluation of a new synthetic brain simulator for endoscopic third ventriculostomy. J Neurosurg Pediatr 2015;15:82-8. [Crossref] [PubMed]
- Rengier F, Mehndiratta A, von Tengg-Kobligk H, et al. 3D printing based on imaging data: review of medical applications. Int J Comput Assist Radiol Surg 2010;5:335-41. [Crossref] [PubMed]
- Tam MD, Laycock SD, Jayne D, et al. 3-D printouts of the tracheobronchial tree generated from CT images as an aid to management in a case of tracheobronchial chondromalacia caused by relapsing polychondritis. J Radiol Case Rep 2013;7:34-43. [Crossref] [PubMed]
- Tam MD, Laycock SD, Bell D, et al. 3-D printout of a DICOM file to aid surgical planning in a 6 year old patient with a large scapular osteochondroma complicating congenital diaphyseal aclasia. J Radiol Case Rep 2012;6:31-7. [Crossref] [PubMed]
- Vakharia VN, Vakharia NN, Hill CS. Review of 3-dimensional printing on cranial neurosurgery simulation training. World Neurosurg 2016;88:188-98. [Crossref] [PubMed]
- Zheng YX, Yu DF, Zhao JG, et al. 3D printout models vs. 3D-rendered images: Which is better for preoperative planning? J Surg Educ 2016;73:518-23. [Crossref] [PubMed]
- Atalay HA, Ulker V, Alkan I, et al. Impact of three-dimensional printed pelvicaliceal system models on residents’ understanding of pelvicaliceal system anatomy before percutaneous nephrolithotripsy surgery: a pilot study. J Endourol 2016;30:1132-7. [Crossref] [PubMed]
- Barber SR, Kozin ED, Dedmon M, et al. 3D-printed pediatric endoscopic ear surgery simulator for surgical training. Int J Pediatr Otorhinolaryngol 2016;90:113-8. [Crossref] [PubMed]
- Mashiko T, Konno T, Kaneko N, et al. Training in brain retraction using a self-made three-dimensional model. World Neurosurg 2015;84:585-90. [Crossref] [PubMed]
- Mashiko T, Otani K, Kawano R, et al. Development of three-dimensional hollow elastic model for cerebral aneurysm clipping simulation enabling rapid and low cost prototyping. World Neurosurg 2015;83:351-61. [Crossref] [PubMed]
- Mehta S, Byrne N, Karunanithy N, et al. 3D printing provides unrivalled bespoke teaching tools for autologous free flap breast reconstruction. J Plast Reconstr Aesthet Surg 2016;69:578-80. [Crossref] [PubMed]
- Burdall OC, Makin E, Davenport M, et al. 3D printing to simulate laparoscopic choledochal surgery. J Pediatr Surg 2016;51:828-31. [Crossref] [PubMed]
- Barsness KA, Rooney DM, Davis LM, et al. Evaluation of three sources of validity evidence for a synthetic thoracoscopic esophageal atresia/tracheoesophageal fistula repair simulator. J Laparoendosc Adv Surg Tech A 2015;25:599-604. [Crossref] [PubMed]
- Ryan JR, Almefty KK, Nakaji P, et al. Cerebral aneurysm clipping surgery simulation using patient-specific 3D printing and silicone casting. World Neurosurg 2016;88:175-81. [Crossref] [PubMed]
- Holt BA, Hearn G, Hawes R, et al. Development and evaluation of a 3D printed endoscopic ampullectomy training model (with video). Gastrointest Endosc 2015;81:1470-5.e5. [Crossref] [PubMed]
- Dhir V, Itoi T, Fockens P, et al. Novel ex vivo model for hands-on teaching of and training in EUS-guided biliary drainage: creation of “Mumbai EUS” stereolithography/3D printing bile duct prototype (with videos). Gastrointest Endosc 2015;81:440-6. [Crossref] [PubMed]
- Wen G, Cong Z, Liu K, et al. A practical 3D printed simulator for endoscopic endonasal transsphenoidal surgery to improve basic operational skills. Childs Nerv Syst 2016;32:1109-16. [Crossref] [PubMed]
- Bow H, He L, Raees MA, et al. Development and implementation of an inexpensive, easily producible, time efficient external ventricular drain simulator using 3-dimensional printing and image registration. Oper Neurosurg (Hagerstown) 2018. [Epub ahead of print].
- Bohl MA, Mooney MA, Repp GJ, et al. The Barrow Biomimetic Spine: fluoroscopic analysis of a synthetic spine model made of variable 3D-printed materials and print parameters. Spine (Phila Pa 1976) 2018;43:E1368-75. [PubMed]
- Bohl MA, Mooney MA, Repp GJ, et al. The Barrow Biomimetic Spine: comparative testing of a 3D-printed L4-L5 Schwab grade 2 osteotomy model to a cadaveric model. Cureus 2018;10:e2491. [PubMed]
- Mattei TA, Frank C, Bailey J, et al. Design of a synthetic simulator for pediatric lumbar spine pathologies. J Neurosurg Pediatr 2013;12:192-201. [Crossref] [PubMed]