Temporary axial rotation stabilization for lumbar disc herniation surgery with the ARO® spinal system: a prospective analysis of safety and clinical efficacy
Introduction
Decompressive surgery has a failure rate of between 25% and 32% based on patient reported improvement in clinical symptoms (1,2). Though many hypotheses have been proposed to explain the failures, Abramovitz reported on the clinical results of more than 400 herniation surgery patients and found that significant back pain was associated with 53% of failures of decompressive surgery (3).
While the underlying etiology of low back pain is not fully understood, a link to abnormal movement has been demonstrated (4-6), especially in axial rotation (7,8). In a clinical study of disc herniation patients, it was found that increased spinal movement in the early post-operative phase and poor clinical outcome are associated (6). Of all possible physiological motions, axial rotation induces the greatest stretch of the collagen fibers of the annulus (9). Hyper-physiological axial rotation may lead to fiber rupture and therefore be one cause of injury to intervertebral disc tissues. Once the intervertebral disc is injured, rotation may further increase (10). Excessive axial rotation has been associated with low back pain by multiple authors. Haughton tested a series of patients using positive discograms to define back pain and compared the axial rotation of symptomatic levels with normal levels (7), finding that the symptomatic levels had more than twice the axial rotation in comparison to the normal. Basques studied 35 patients with recurrent low back pain and measured both flexion/extension movements as well as axial rotation movements, and compared their movements to 64 asymptomatic controls (8). The study found significant changes in movement associated with recurrent low back pain including an increase in axial rotation at the L4/L5 level, as well as a loss of movement in flexion/extension.
Because hypermobility of the spine has been implicated in back pain, preventing or limiting motion may be a way of treating painful spinal conditions. Systems that prevent excessive motion have been developed to alleviate back pain (11). One example is the Graf ligament (Neoligaments, Leeds, UK), which restricted flexion by “locking” the instrumented segment in full lordosis (11). Early clinical results were on par with fusion but it is suspected that the system may have caused lateral canal stenosis by biomechanically shifting physiologic loads to the facets (12). Another example is Dynesys (Zimmer Biomet, Warsaw, IN, USA). It too was developed as a non-fusion alternative to rigid instrumentation for restricting motion in the segment. It has been used in conjunction with nucleotomy for disc herniation, with benefit on long-term result (13). Indeed, the latest long-term data show comparable results to rigid instrumentation for fusion (14), but the rate of adjacent segment disease (ASD) is high (15). Thus, both systems do what they were designed to do (limit motion in the sagittal plane). However, the biomechanics of these systems do not stop degeneration. Indeed, they may rather accelerate it. Thus, their clinical success is likely to remain suboptimal.
Instrumentation to limit only axial rotation is novel. The ARO® Spinal System is designed to provide temporary axial rotation stabilization in a lumbar motion segment where the herniation is present (Figure 1). It is placed on the ipsilateral side as the partial discectomy and herniation. A suture anchor is placed in the pedicle of the lower vertebral body and a button on contralateral side of the spinous process of the upper vertebral body. A high-strength suture tethers the button to the anchor. The suture is lightly tensioned (75N, or approximately 17 pounds) to address ligamentous laxity. Biomechanically, it prevents rotation toward the side of the disc herniation, potentially preventing fiber rupture, further rotation and back pain.
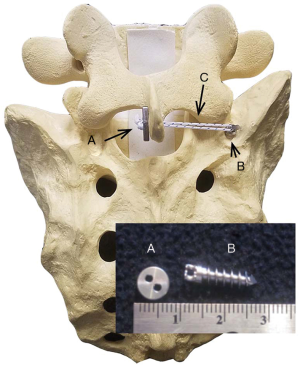
Methods
Study design
Our aim of the current study was to test the safety of this novel dynamic stabilization device system while gathering clinical outcomes data as well. This was a prospective non-randomized, clinical trial, approved by the local ethic committee (Aarhus University Hospital, Denmark, Project ID: M-20100224), Danish Medicines Agency record number 2013033802 and registered at www.ClinicalTrials.gov (ID: ARO-01).
Participants
Included patients were treated at the Aarhus University Hospital orthopedic outpatient clinic after being referred by a general practitioner. Potential participants had to be between 18 and 55 years of age at time of consent.
Inclusion criteria were radicular pain and evidence of nerve-root irritation lasting 6 weeks or more as evidenced by both radicular pain below the knee and sign of nerve root irritation. Positive straight leg raises, femoral tension sign, or neurologic deficit was considered sign of nerve root irritation. Potential participants had a primary one-level posterolateral herniation in the lower lumbar spine (L4-L5 or L5–S1 only) as identified by magnetic resonance (MR) imaging (protrusion, extrusion or sequestered fragment) consistent with the clinical symptoms (both level and side). If clinical indications for surgery were met, patients were invited to participate in the clinical trial and to receive a discectomy and the ARO implant.
Exclusion criteria were previous lumbar surgery, cauda equina syndrome, scoliosis greater than 15 degrees, osteoporosis, segmental instability evaluated by biplanar X-ray (>10 degrees angular motion or >4 mm translation), vertebral fractures, spinal infections, spinal tumors, and inflammatory spondyloarthropathy.
Outcome measurements
Safety was documented in terms of adverse events and device-related adverse device effects. These are defined by ICH Harmonized Tripartite Guidelines as death, life threatening condition, hospitalization or prolonging of hospitalization, and persistent or significant disability/incapacity. Anterior-posterior and medial-lateral X-rays were obtained at 6 and 12 months postoperatively and evaluated for safety. Estimated blood loss, surgery time, dural tears, dural hematoma, and post-operative infections were also recorded.
Additional outcomes were patient-reported visual analog scales (VAS) for leg and back pain, Oswestry Disability Index (ODI) outcomes, and pain medication. General health status was gathered using the EuroQol 5D (EQ-5D). Patients were also asked how satisfied they were with the result, and if they would choose same treatment or differently. Follow-up was done at 6, 12 weeks, 6 and 12 months. Results were compared with standard discectomy from the literature.
Statistical methods
Due to the fact that data were not normally distributed, tendencies for leg and back pain VAS scores, ODI and EQ5D are stated as median scores. For test of significance Wilcoxon signed-rank test were performed. For nominal data (sick leave and pain medication) McNemar’s test of significance was used. The level of significance was set at P<0.05.
Results
Study enrollment and follow-up
The study enrollment period lasted from April 2012 to April 2014. A total of 179 patients were screened; 154 did not meet the inclusion criteria (either because of improvement after being referred from the general practitioner (GP) or because the patient chose to continue conservative treatment instead of undergoing surgery). The remaining 25 patients met the inclusion/exclusion criteria except three patients who were not interested in experimental surgery and two patients who had surgery in another clinic, leaving a total of 20 patients to be included. Follow-up ended April 2015 at 1 year after surgery. All patients completed the final follow-up period. Although two patients missed the 6-week follow-up, all did complete the 12-, 25- and 52-week follow-ups.
Baseline data
Patient demographics are shown in Table 1. Ten participants were female and 10 were male. The average age was 38 years (range, 24–54 years). Smokers and past smokers represented 75% of the study population. The great majority of participants (80%) were employed at the time of surgery but more than half of the hole cohort were on sick leave (58%). Nevertheless, most participants exercised to some degree (75%). Most patients had combined leg and back pain (84%) for minimum of 3 months and a maximum of 12 months; 16% had only leg pain. Almost all patients were using painkillers (95%); of these, half were using opioids (50%). Most patients had herniation on level L5–S1 (90%).
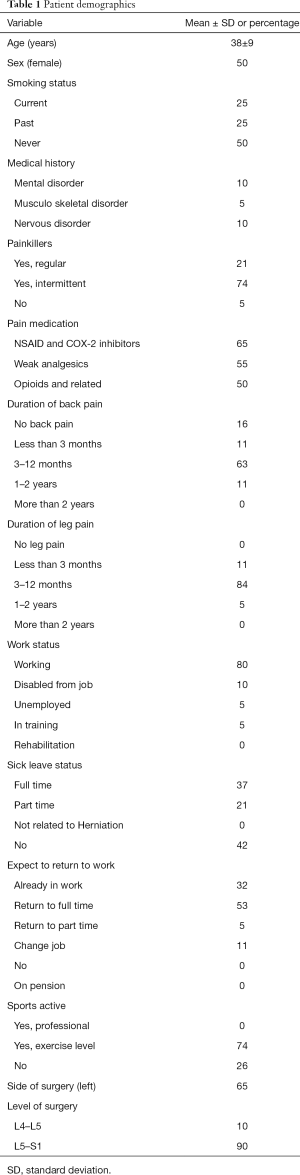
Full table
Medical and surgical results
Surgeries lasted 89±15 minutes with an average estimated blood loss of 60±26 mL.
Adverse events
There were no complications with the surgery and only four serious adverse events were reported in four different patients, none related to the device. The most serious was a patient who had re-operation with instrumented fusion 3 months after primary operation due to unrelenting pain. The patient had severe disc degeneration at the affected level in both the pre-operative and MRs at follow-up. At the surgery, the ARO device was removed. At 1 year follow-up, the patient reported continuing back pain and radiculopathy in right leg, although improved. It was adjudged that the device did not cause this complication as the pain was believed to be associated with the disc degeneration, which predated the implantation of the device.
The other three serious adverse events were medical complications. One was a prolonged hospital admission (1 day) in a diabetic patient who got hypoglycemic after surgery. Another patient got cholecystolithiasis 6 months after surgery; the patient was admitted to hospital and treated with antibiotics. The last adverse event was an ileus in a patient, 9 months after inclusion, was also admitted to hospital and treated conservatively with success. None of the 20 patients had common complications such as post-surgery wound infection, dural tears or dural hematoma. X-rays were used to evaluate implant position; no breakage or loosening were seen. There were no device-related adverse events.
Functional outcomes
VAS leg and back pain scores are enumerated in Table 2. The median VAS leg pain score decreased from 56 pre-operatively to 3 at 1 year (P=0.01). The median VAS back pain score likewise decreased from 35 pre-operatively to 6 at 1 year (P=0.04). ODI showed a significant decrease (P<0.01), from a pre-operative median score of 38 to 5, 1 year post-operatively, Table 2. The EQ-5D scores are listed in Table 2. Both EQ VAS and EQ-5D indices showed a significant improvement from pre-operative to 1 year follow-up (P<0.01).
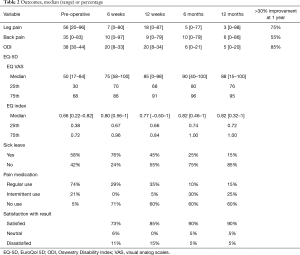
Full table
When asked if the patient was satisfied with the result of surgery, 90% answered yes, 5% were in doubt, and 5% answered no. Before surgery 58% were on sick leave, this had changed to 76% at 6 weeks, 45% at 12 weeks, 25% at 6 months and 15% at 12 months (P<0.01), Table 2.
Patients were also asked if they took pain medication. After 1 year 60% did not use any form of analgesics, 15% used them regularly and 25% used them intermittently (P<0.01); only 15% used opioids.
Discussion
The ARO spinal system proves equally safe to standard discectomy when compared to the literature. There was one reoperation (5%) and no wound infections, no dural tears, and no dural hematomas. These results compare favorably to those of the SPORT study (16), which reported a 4% reoperation rate, 2% wound infections and 4% dural tears.
One patient with unrelenting pain was re-operated. An additional MR scan showed severe degeneration in the afflicted disc. It was the adjudged cause of the ongoing pain and the patient received an instrumented lumbosacral fusion. Reoperation after discectomy is more commonly performed due to reherniation (17), but this was not found during the follow-up period.
One factor that increases the risk of infection is longer surgery time. No post-operative wound infections occurred in this study. This is possibly related to the relatively short surgery time. The surgery time was negatively impacted by the relative simplicity of the surgical instruments provided. Improved instrumentation would be expected to reduce the surgery time.
Because the ARO Spinal System is implanted relatively far from both the dura and the nerve roots, we did not expect to see any complications involving neural structures. This was confirmed in the study, as none of the patients undergoing surgery with the ARO device had dural tears or dural hematoma. These results compare favorably to those of the SPORT study (16), which reported 4% dural tears.
Patients reported significant improvements in pain score with surgery and the ARO implant, numerically superior to, but not statistically superior to standard herniated lumbar surgery without use of an implant. Leg pain is the reason to undergo surgery and a remarkable improvement is expected here. The Swedish Spine Registry (18) reports mean VAS leg pain scores of 67 pre-operatively and 22, 1 year post-operatively or a change of 45 points; in this study the median VAS leg pain diminished from 56 pre-operative and to 3 over the same period, a change of 53. In the Swedish Spine Registry (18) there is improvement from a mean pre-operative VAS back pain score of 46 to 26 post-operatively, a change of 20. Likewise, the patients in this study had a pre-operative median VAS back pain score of 35 and a post-operative VAS score of 6, a change of 29.
EQ5D and ODI, both validated questionnaires (19,20), were used to measure progression in health and disability. Both showed improvement from pre-operative to 12 months postoperatively. A very large percentage, 90% was satisfied with their result, including the patient who had reoperation. In comparison only 78% were satisfied in the Swedish Spine register (18).
Narrow inclusion criteria were implemented, such as no prior lumbar spine surgery, no spinal deformity, and only posterolateral herniation. This resulted in a homogeneous study group. It is easier to explain complications or severe adverse events (SAE) with homogeneous study groups because such groups have less concurrent comorbidity. Unfortunately, it also resulted in the exclusion of many potential candidates with disc herniation and extended the study period. On the other hand, one advantage of the study design was that only one surgeon performed all the procedures. This avoided some of the variability that occurs in studies with multiple investigators with varying treatment patterns.
With this relative short-term follow-up, we did not anticipate adjacent segment degeneration, as this appears in the longer-term (17). By limiting only unilateral rotation we believe that adjacent segment degeneration will be delayed, although future follow-up on this trial should evaluate this.
One limitation of this study is the usability of the effect measures. This small a group is suitable for observation of SAE but not as suited for the evaluation of a treatment effect. For this, a large randomized clinical trial (RCT) should be conducted. Randomizing the subjects and blinding the data will prevent methodological errors. A larger number of subjects treated will reveal if there is clinical significance for the patient, which is different from statistical significance. Alternatively, more detailed comparisons to registry data might provide a means to evaluate the treatment effect.
To facilitate interpretation, the minimal clinical important difference (MCID) has been proposed to analyze outcome (21). While the methodology for MCID is not completely established, it has been suggested that 30% reduction in self-report back pain measures is a suitable MCID (22). In comparison, this study found that most subjects (18/20) had a more than 30% improvement in either leg or back pain. Only two subjects reported no improvement, or less than 30%, in both leg pain and back pain. These are also the same two subjects who are not satisfied with the result, when asked at the end of study. One of these patients had the reoperation. Therefore, it appears that patient dissatisfaction correlates with insignificant improvement in both leg and back pain (unsurprisingly) and patient satisfaction comes with significant improvement in either leg or back pain, even though the main purpose for surgery is to relieve leg pain.
In conclusion, when discectomy was combined with the ARO Spinal System to treat symptomatic lumbar disc herniation, patients had significant improvement in clinical outcomes and the system had a benign safety profile. Further research is required to determine if the system provides benefits beyond treatment by discectomy alone.
Acknowledgements
Funding: ARO Medical ApS has sponsored the study. Danish Council for Strategic Research grant (0603-00212B) funds were received in support of this work.
Footnote
Conflicts of Interest: Dr. Cody Bünger reports grants, personal fees, non-financial support and other from University of Aarhus, grants from “Spydspidspulje” (Public funded by the hospital) for growth rod project, grants from Danish Strategic Research Foundation, grants from Mærsk Foundation, grants from Danish Rheumatism Association, grants from Danish Regional Fund, personal fees from Stryker, personal fees from Globus Medical, personal fees from Medtronic, personal fees from DePuy Synthes, outside the submitted work; and hold global patents for early-onset scoliosis instrumentation, not applied in the study. Bruce Robie reports ownership interest in ARO Medical. Other authors have no conflicts of interest to declare.
Ethical Statement: This was a prospective non-randomized, clinical trial, approved by the local ethic committee (Aarhus University Hospital, Denmark, Project ID: M-20100224), Danish Medicines Agency record number 2013033802 and registered at www.ClinicalTrials.gov (ID: ARO-01).
References
- Weinstein JN, Lurie JD, Tosteson TD, et al. Surgical versus nonoperative treatment for lumbar disc herniation: four-year results for the Spine Patient Outcomes Research Trial (SPORT). Spine 2008;33:2789-800. [Crossref] [PubMed]
- Tosteson AN, Lurie JD, Tosteson TD, et al. Surgical treatment of spinal stenosis with and without degenerative spondylolisthesis: cost-effectiveness after 2 years. Ann Intern Med 2008;149:845-53. [Crossref] [PubMed]
- Abramovitz JN, Neff SR. Lumbar disc surgery: results of the Prospective Lumbar Discectomy Study of the Joint Section on Disorders of the Spine and Peripheral Nerves of the American Association of Neurological Surgeons and the Congress of Neurological Surgeons. Neurosurgery 1991;29:301-7; discussion 307-8. [Crossref] [PubMed]
- Fujiwara A, Tamai K, An HS, et al. The relationship between disc degeneration, facet joint osteoarthritis, and stability of the degenerative lumbar spine. J Spinal Disord 2000;13:444-50. [Crossref] [PubMed]
- Iguchi T, Kanemura A, Kasahara K, et al. Lumbar instability and clinical symptoms: which is the more critical factor for symptoms: sagittal translation or segment angulation? J Spinal Disord Tech 2004;17:284-90. [Crossref] [PubMed]
- Halldin K, Zoega B, Karrholm J, et al. Is increased segmental motion early after lumbar discectomy related to poor clinical outcome 5 years later? Int Orthop 2005;29:260-4. [Crossref] [PubMed]
- Haughton VM, Rogers B, Meyerand ME, et al. Measuring the axial rotation of lumbar vertebrae in vivo with MR imaging. AJNR Am J Neuroradiol 2002;23:1110-6. [PubMed]
- Basques BA, Espinoza Orías AA, Shifflett GD, et al. The Kinematics and Spondylosis of the Lumbar Spine Vary Depending on the Levels of Motion Segments in Individuals with Low Back Pain. Spine 2017;42:E767-74. [Crossref] [PubMed]
- Schmidt H, Kettler A, Heuer F, et al. Intradiscal pressure, shear strain, and fiber strain in the intervertebral disc under combined loading. Spine (Phila Pa 1976) 2007;32:748-55. [Crossref] [PubMed]
- Oxland TR, Crisco JJ 3rd, Panjabi MM, et al. The effect of injury on rotational coupling at the lumbosacral joint. A biomechanical investigation. Spine (Phila Pa 1976) 1992;17:74-80. [Crossref] [PubMed]
- Mulholland RC, Sengupta DK. Rationale, principles and experimental evaluation of the concept of soft stabilization. Eur Spine J 2002;11 Suppl 2:S198-205. [PubMed]
- Grevitt MP, Gardner AD, Spilsbury J, et al. The Graf stabilisation system: early results in 50 patients. Eur Spine J 1995;4:169-75; discussion 35. [Crossref] [PubMed]
- Putzier M, Schneider SV, Funk JF, et al. The surgical treatment of the lumbar disc prolapse: nucleotomy with additional transpedicular dynamic stabilization versus nucleotomy alone. Spine (Phila Pa 1976) 2005;30:E109-14. [Crossref] [PubMed]
- Hoppe S, Schwarzenbach O, Aghayev E, et al. Long-term Outcome After Monosegmental L4/5 Stabilization for Degenerative Spondylolisthesis With the Dynesys Device. Clin Spine Surg 2016;29:72-7. [Crossref] [PubMed]
- St-Pierre GH, Jack A, Siddiqui MM, et al. Nonfusion Does Not Prevent Adjacent Segment Disease: Dynesys Long-term Outcomes With Minimum Five-year Follow-up. Spine (Phila Pa 1976) 2016;41:265-73. [Crossref] [PubMed]
- Weinstein JN, Tosteson TD, Lurie JD, et al. Surgical vs nonoperative treatment for lumbar disk herniation: the Spine Patient Outcomes Research Trial (SPORT): a randomized trial. JAMA 2006;296:2441-50. [Crossref] [PubMed]
- Hilibrand AS, Robbins M. Adjacent segment degeneration and adjacent segment disease: the consequences of spinal fusion? Spine J 2004;4:190s-4s. [Crossref] [PubMed]
- Strömqvist B, Fritzell P, Hägg O, et al. Swespine: the Swedish spine register: The 2012 report. Eur Spine J 2013;22:953-74. [Crossref] [PubMed]
- Fairbank JC, Pynsent PB. The Oswestry Disability Index. Spine (Phila Pa 1976) 2000;25:2940-52; discussion 52. [Crossref] [PubMed]
- Rabin R, de Charro F. EQ-5D: a measure of health status from the EuroQol Group. Ann Med 2001;33:337-43. [Crossref] [PubMed]
- Copay AG, Subach BR, Glassman SD, et al. Understanding the minimum clinically important difference: a review of concepts and methods. Spine J 2007;7:541-6. [Crossref] [PubMed]
- Dworkin RH, Turk DC, Farrar JT, et al. Core outcome measures for chronic pain clinical trials: IMMPACT recommendations. Pain 2005;113:9-19. [Crossref] [PubMed]